The benefits of genetically modified organisms
“Genetically modified organisms,” are frequently the subject of controversy, mostly because they are misunderstood. Humans have been genetically modifying plants and animals for millennia. New methods hold the potential to cure horrible diseases and make food more nutritious, potentially improving millions of lives.
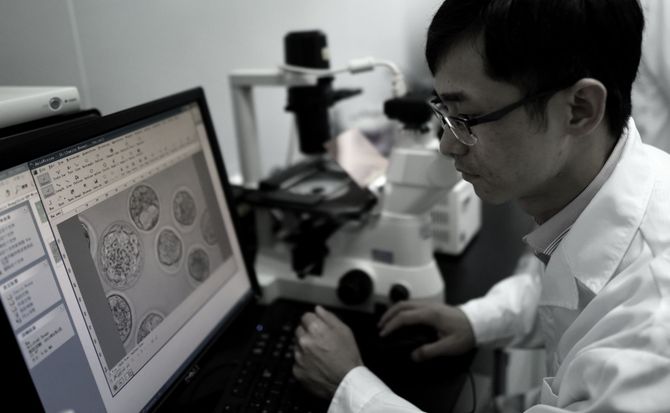
In a nutshell
- Much of the criticism of GMOs is ill-informed
- Recent advances offer hope for huge benefits
- Regulation must be toned down for such solutions to be fully exploited
This opinion was written by Dr. Therese Liechtenstein and Dr. Henry I. Miller
The issue of so-called “genetically modified organisms,” or GMOs, is frequently in the news and the subject of controversy. Perhaps pseudo-controversy is a better term, because much of the criticism is gratuitous and ill-informed. Genetic modification is by no means new; in fact, over the course of human history we have continuously genetically modified animals and plants through selection and breeding to enhance their desirable characteristics. Humans have invented increasingly sophisticated techniques, particularly since the discovery that all higher organisms have the same carrier of genetic information, the double-stranded helix of DNA.
Since the advent in the 1970s of recombinant DNA technology, which enables segments of DNA to be moved readily and more precisely from one organism to another, molecular genetic engineering techniques have become ever more sophisticated, precise and predictable. This evolution has now culminated in the most recent discoveries, the CRISPR-Cas9 system and base editing.
CRISPR (short for Clustered Regularly Interspaced Short Palindromic Repeats) is a natural defense system that a range of bacteria use against invading viruses. CRISPR can recognize specific DNA sequences, while the enzyme Cas9 cuts the DNA at the recognized sequence. As often happens in science – and reminiscent of mutagenesis a century ago and recombinant DNA technology in the 1970s – molecular biologists and genetic engineers quickly copied and adapted the naturally occurring system. Using CRISPR-Cas9, scientists can target and edit DNA at precise locations, deleting, inserting or modifying genes in microorganisms, plants and animals, including humans. CRISPR-Cas9 is cheaper, faster, easier, more precise and more predictable than its genetic engineering predecessors, and scientists are continuously improving the technique, its predictability and safety.
Genetic revolution
CRISPR-Cas9 presages a revolution in agriculture and human medicine because it is so much more precise and predictable than earlier techniques. Precision and predictability are important to ensure that the results are safe and achieve their desired ends. There are notable historical examples of older, pre-molecular techniques of genetic modification in agriculture that turned out to be missteps. Examples include the Lenape potato, which contained elevated, harmful levels of a plant alkaloid; the creation of hyper-aggressive Africanized honeybees by crossbreeding African and European species in the 1950s; and inadvertently making some varieties of corn in the United States more susceptible to the Southern Corn Leaf Blight fungus, which resulted in significant crop losses in 1970.
Precision and predictability are especially critical in human gene therapy.
Precision and predictability are especially critical in human gene therapy. Previous clinical trials have shown impressive results, for example, in treating children with the X-linked severe combined immunodeficiency (SCID) disorder, also known as “bubble boy disease.” These patients’ defective immune system makes them extremely vulnerable to infectious diseases, so that to survive they must live in a sequestered, sterile environment.
However, using a gene therapy technique that was less precise than CRISPR-Cas9, some children, although cured of their genetic disease, developed cancer. Since then, researchers have searched for more precise techniques – and CRISPR-Cas9 and its further refinements may be the answer.
CRISPR was only discovered in 2012, but since then has been quickly adopted by research laboratories worldwide and is already being used in clinical trials. These studies focus primarily on cancer patients, where immune cells are modified outside of the patient (ex vivo) and returned, to better destroy cancer cells. But scientists and companies are already working on using CRISPR-Cas9 and other analogous techniques for treating, curing, or preventing other diseases.
Many diseases are caused by defective genes and are therefore amenable to treatment by replacing or repairing faulty genes. The company CRISPR Therapeutics (founded by CRISPR co-inventor Dr. Emmanuelle Charpentier) is targeting beta thalassemia, an inherited blood disease in which the bone marrow does not produce enough healthy red blood cells. Clinical trials are planned to start this year. (A study using a different gene therapy approach showed great promise against this disease in a clinical trial published in April of this year.)
A competitor, Editas Medicine (founded by the other CRISPR inventor, Dr. Jennifer Doudna) is focusing on a rare form of congenital blindness that causes severe vision loss at birth, and is also planning to start clinical trials this year. Other diseases that are being targeted by researchers include sickle-cell anemia, Duchenne muscular dystrophy and HIV.
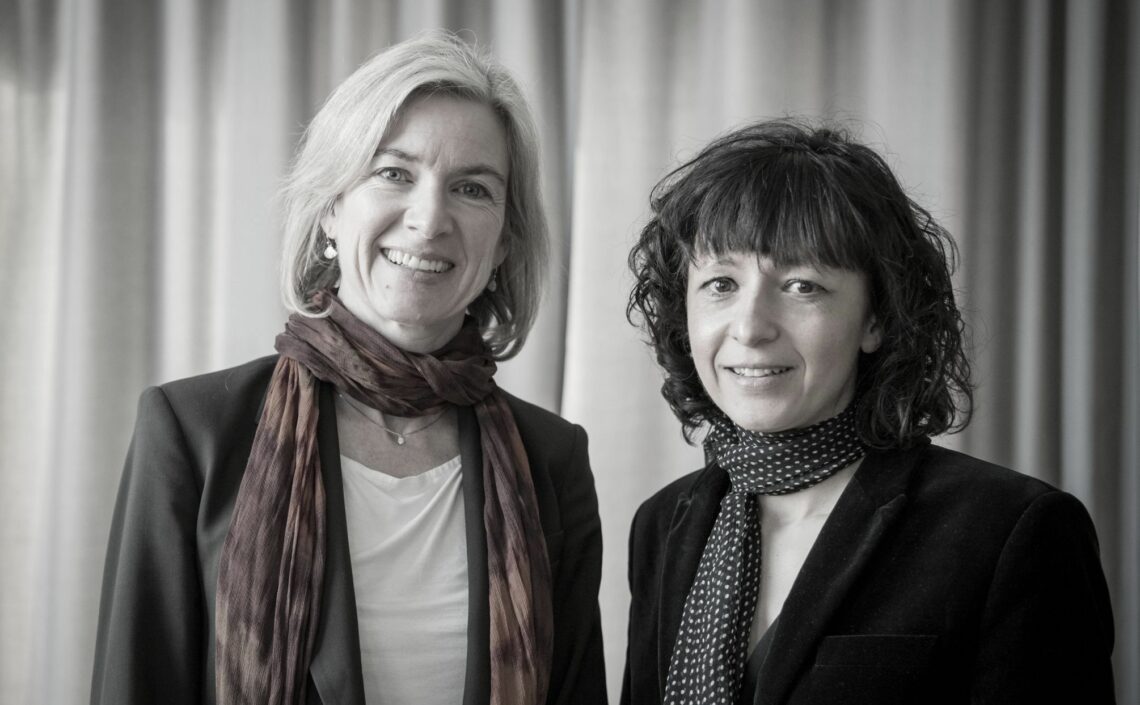
These attempts at gene therapy are intended to correct a gene within specific cells in the body, which means that not all the cells of the patient will contain the corrected DNA; and if the modified cells are not egg or sperm cells or embryos, the genetic changes are not passed on to subsequent generations. This “somatic cell gene therapy” has not engendered significant controversy.
Germline correction
More controversial is germline gene editing, changing genes within eggs, sperm or embryo cells, since those changes would be passed on to future generations. Nevertheless, preclinical research using CRISPR-Cas9 to make germline modifications is advancing rapidly.
A major advance came in August 2017, when a team led by Shoukhrat Mitalipov of Oregon Health & Science University in Portland used CRISPR-Cas9 to correct a disease-causing mutation in dozens of viable human embryos for the first time. The researchers corrected a mutation in gene MYBPC3, which causes hypertrophic cardiomyopathy and can lead to sudden heart failure and death. The scientists grew the corrected embryos until the blastocyst stage, but did not implant them into a woman’s uterus, which would be prohibited in the U.S. In other countries, such as Germany and Austria, legislation would not have even allowed the experiment in the first place.
The modified human embryos displayed normal development, making it tempting to assume that they could have developed into viable fetuses. Although the experiment demonstrated remarkable efficiency without off-target effects, the researchers concluded that “genome editing approaches must be further optimized before clinical application of germline correction can be considered.” The field is still in its infancy and most researchers agree that further testing is needed. In addition, other research groups have raised questions about the veracity of the Mitalipov group’s interpretation of the data, a reminder that a substantial amount of research still must be performed before germline correction should be applied in humans.
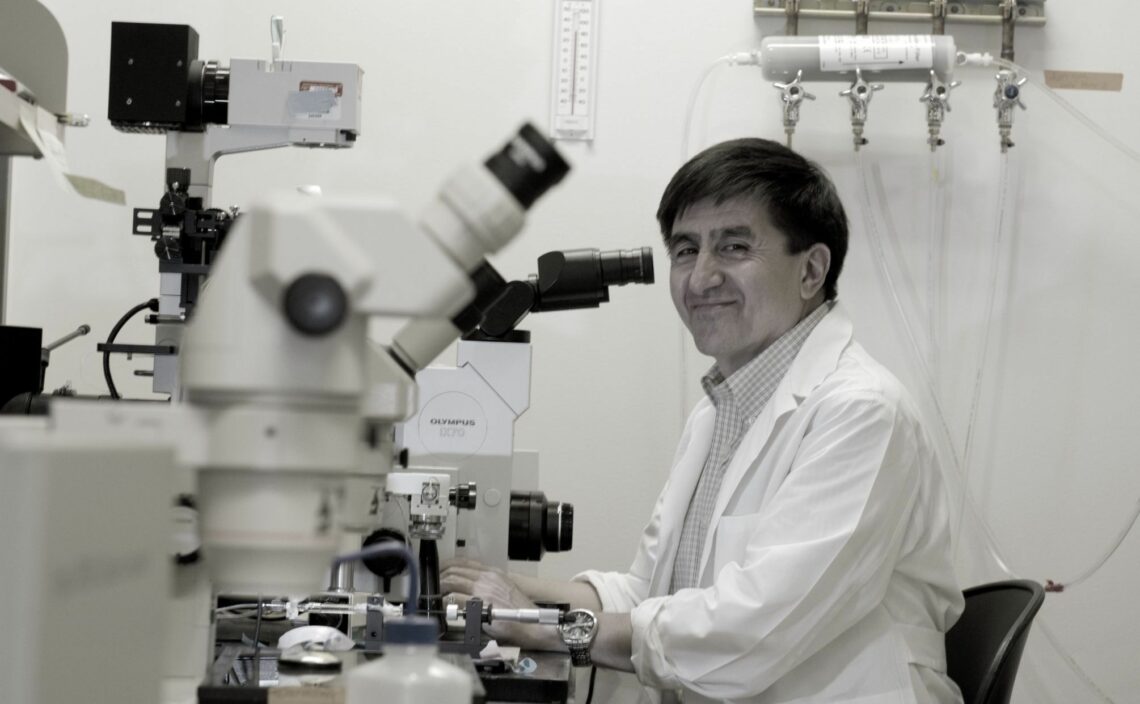
Correcting defective genes in human embryos has the potential to alleviate severe diseases. Instead of discarding embryos with faulty genes (as is done during in vitro fertilization today), one could use CRISPR to correct them. Such therapy – if allowed in the future – would likely be as heavily regulated as new drugs, overseen by governmental agencies and ethics committees. And although concerns have been raised by ethicists about the creation of embryos with “enhanced” traits that give rise to so-called “designer babies,” it is unthinkable that regulators or research institutions’ review boards would approve genetic engineering of embryos in the absence of a demonstrated medical need.
But what about countries where governments and regulatory agencies may not be as strict or pursue their own agendas? There are fears that a tool like CRISPR-Cas9 could lead us down a path of breeding super-humans, super-soldiers, docile citizens (as in Aldous Huxley’s dystopian Brave New World) and so on.
For the foreseeable future, this fear is misplaced. While we have identified defective genes that cause many diseases, we do not yet understand which genes are linked to intelligence, docility or other complex traits. Such attributes are probably the result of a combination of many genes (as well as epigenetic and environmental factors) and we are still far away from being able to create humans with desired, complex character traits. Moreover, it is unlikely that clinical trials to achieve these sorts of “enhancements” would be approved by regulators (at least in Western democracies), similar to the situation for “designer babies” discussed above.
Overregulation
Another potentially revolutionary genetic engineering innovation almost ready for the clinic is xenotransplantation, the transplanting of animal organs into humans. Improved immunosuppressant drug regimens and increasing numbers of pig lines that have been gene-edited to eliminate antigens that would cause rejection by the human recipient are a potential game-changer. The experiments in which porcine organs have been transplanted into monkeys are very promising. There will likely be a day when xenotransplantation will reduce the vast number of people on waiting lists for organs.
Critics’ objections often fail to assess the costs of not undertaking such therapeutic initiatives.
Critics of such cutting-edge genetic techniques sometimes invoke the so-called Precautionary Principle to call for delays, but their objections often fail to assess the costs of not undertaking such therapeutic initiatives.
The same holds true for applications to agriculture. Farmers and plant breeders have been selecting and hybridizing plants to enhance their desirable characteristics for millennia. Modern varieties of innumerable crop plants such as corn, tomatoes and wheat now bear little resemblance to their ancestors. A common technique for creating new plant varieties, which originated about a century ago, is to subject seeds to radiation to scramble their DNA and create mutants, some of which may (and often do) exhibit desirable traits. Thousands of plant varieties that are consumed routinely – including lettuce, wheat, rice, oats and the popular Ruby-Sweet and Rio Star pink grapefruit varieties – were derived this way.
With the advent of molecular techniques – first recombinant DNA technology and, more recently, CRISPR-Cas9, base editing and so on – we can transfer or alter a single gene in a controlled manner.
Thus, there is a seamless continuum of techniques for genetically modifying of organisms. Yet, the older, less precise, less predictable techniques have not elicited concerns from regulators or activists, and crops modified with those more primitive technologies are not as heavily regulated as those modified with the more precise techniques. This turns on its head a basic tenet of regulation – that the degree of regulation should be commensurate with the perceived risk. Sui generis regulation of products made with molecular genetic modification techniques abjectly and consistently fails cost-benefit analyses: There are prodigious costs, but no benefits.
Various academic groups have proposed approaches that would remedy the regulatory distortion. For example, in 2016 Dr. Henry I. Miller (one of the authors of this report) with his colleagues published an article that described a risk-based approach to the regulation of organisms genetically engineered by any technique, that would make regulation scientifically defensible and risk-based. Using this approach, the extent of regulatory review would be based on the characteristics of the product, not the process by which it is obtained. The risk of the parental organism and the function of the gene introduced or altered would determine the degree of regulation required. Currently, lengthy (and arguably unnecessary) case-by-case reviews are required for many products made with molecular techniques, which boosts research and development costs and hinders progress.
Moral imperative
Genetic engineering of crops can enhance crop yields and nutritional quality, increase resistance to pests, improve tolerance to drought and reduce insecticide use. Biofortified crops in particular can have a significant impact, especially for people in the developing world who obtain most of their calories from one or a small number of subsistence crops that may be deficient in essential vitamins or minerals. One example is “Golden Rice,” fortified with beta-carotene, the precursor of vitamin A, whose consumption can prevent vitamin A deficiency. (Vitamin A deficiency is common in places where people obtain most of their calories from rice, which does not contain the compound.)
There is no reason to fear technologies that are more precise and predictable than their predecessors.
The World Health Organization estimates that about 250 million preschool-aged children are affected by vitamin A deficiency, the leading cause of preventable blindness of children in developing countries. Up to 500,000 vitamin A deficient children are estimated to become blind each year, with half of them dying within 12 months of losing their sight. Astonishingly, Golden Rice has recently been approved in Australia and Canada, but because of opposition from activists and inaction from regulators, not in countries that desperately need it to combat vitamin A deficiency.
A report from the Pontifical Academy of Sciences on “transgenic plants for food security in the context of development” in 2009 concludes that it is a “moral imperative to make the benefits of [genetic engineering] technology available on a larger scale to poor and vulnerable populations … to raise their standards of living, improve their health and protect their environments.” The report points out that more than a billion people are currently undernourished and that the risk of inaction must also be considered.
Molecular techniques such as CRISPR-Cas9 and base editing are the most advanced techniques currently available in our quest to alter organisms in a precise and predictable fashion for commercial and humanitarian advances. They could be used to improve the lives of millions of patients with untreatable diseases, combat hunger and malnutrition, protect the environment through decreased use of insecticides, and much more. There is no reason to fear technologies that are more precise and predictable than their predecessors. In their risk analyses, policymakers and end-users need to include the consequences of not using new products, processes and technologies.
The fear of gene editing in humans is reminiscent of the fear that was widespread when vaccination was introduced in the 19th century. Most of those fears have vanished into the mists of history, and vaccines have enabled us to eradicate smallpox and make tremendous inroads toward reducing many other diseases, especially serious childhood viral illnesses.
We expect that widespread public acceptance will also come to gene editing as, over time, successes accrue to applications in agriculture, environmental protection, medicine and other fields. As Max Planck, the German theoretical physicist and Nobel laureate, observed, “A new scientific truth does not triumph by convincing its opponents and making them see the light, but rather because its opponents eventually die, and a new generation grows up that is familiar with it.”
Gene editing will be today’s scientists’ legacy to future generations.
Dr. Therese Liechtenstein, a biologist who worked with gene editing techniques during her PhD in Immuno-Oncology at University College London, is managing strategic projects at the Healthcare Strategy Realization Office of Merck. This article does not reflect the views of Merck and does not relate to any work or products of the company.
Dr. Henry I. Miller, a physician and molecular biologist, is the Robert Wesson Fellow in Scientific Philosophy and Public Policy at Stanford University’s Hoover Institution. Dr. Miller served for 15 years at the U.S. Food and Drug Administration (FDA) in a number of posts. He was the medical reviewer for the first genetically engineered drugs to be evaluated by the FDA.